SDS-PAGE
Related articles
Secondary antibodies resources
Alexa Fluor secondary antibodies
Biotinylated secondary antibodies
Enhancing Detection of Low-Abundance Proteins
9 tips for detecting phosphorylation events using a Western Blot
Western Blotting with Tissue Lysates
Immunohistochemistry introduction
Immunohistochemistry and Immunocytochemistry
Immunohistochemistry troubleshooter
Chromogenic and Fluorescent detection
Preparing paraffin-embedded and frozen samples for Immunohistochemistry
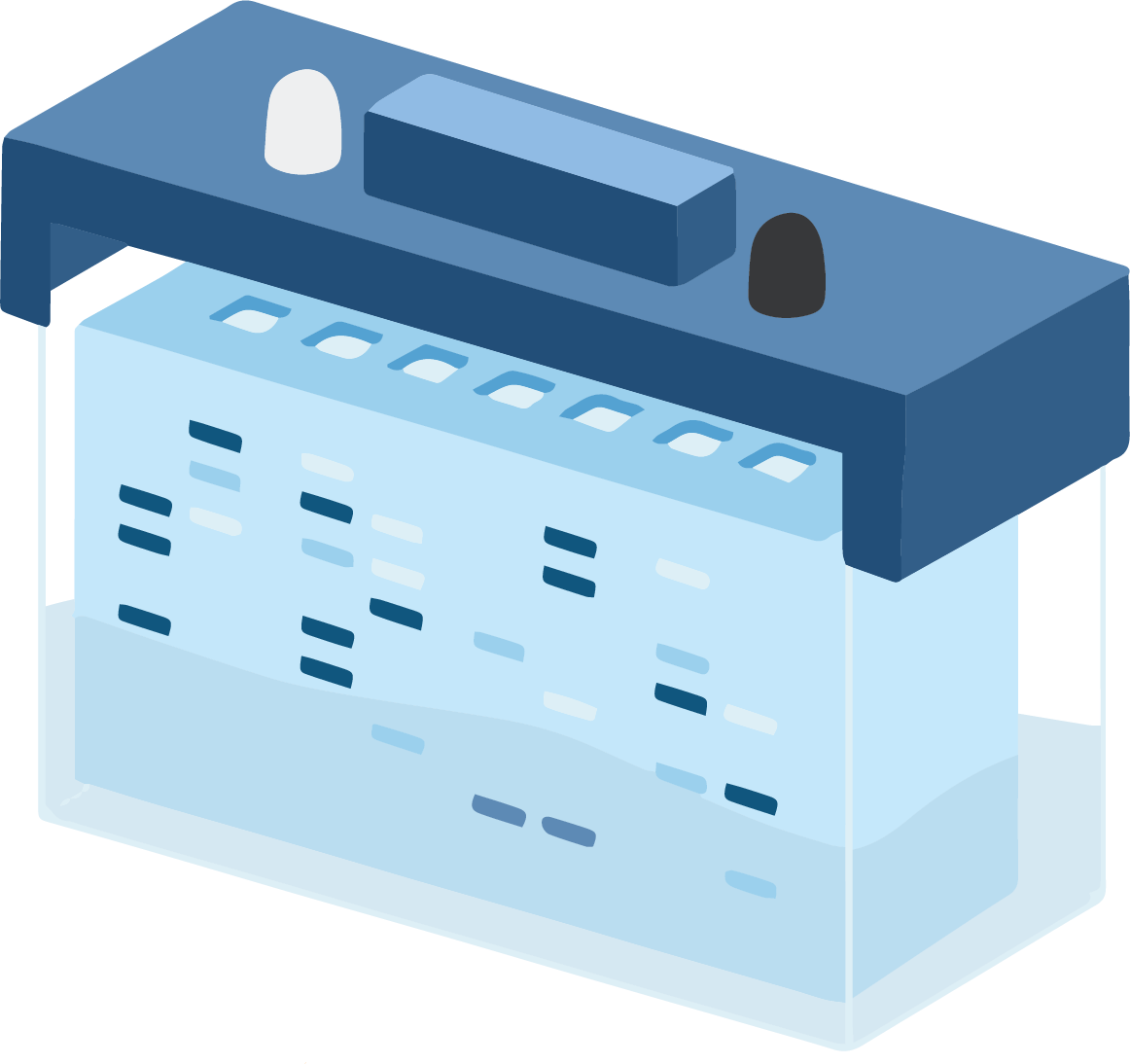
Sodium Dodecyl Sulphate - Polyacrylamide Gel Electrophoresis
SDS-PAGE (or Sodium Dodecyl Sulphate–Polyacrylamide Gel Electrophoresis in its long form), is a commonly used process which separates proteins out by their molecular masses. The use of the two compounds - SDS and Polyacrylamide - are key components in the separation of proteins by mass alone.
By applying a ubiquitous negative charge across protein structures the proteins can move through a polyacrylamide gel matrix from one electrode towards another - independently of charge and are separated solely on the basis of differences in their molecular weight.
A typical SDS-PAGE might be used to separate proteins between 5 kDa to 250 kDa (kiloDaltons).
SDS-PAGE Apparatus
The SDS-PAGE process uses a specialist piece of kit, which uses simple design features. This consists of a chamber in which a smaller chamber is placed.
The smaller chamber holds up the gel cassettes, which sandwich firmly together. This effectively results in a bucket within a bucket type scenario, whereby the smaller inner chamber is filled with one buffer, and the outer chamber is filled with a separate buffer.
The kit is normally set up so that the inner chamber buffer makes contact between the stacking gel and cathode, and the outer chamber makes contact between the running gel and the anode.
SDS plays an important role in the process, acting as a surfactant which masks the intrinsic charges found within the quarternary structure of proteins and applying a uniform negative charge across proteins, which ensures the proteins are able to move through the polyacrylamide gel according to their mass.
The amphipathic nature of SDS also means it can unfold both polar and non-polar regions of a protein's structure, thus dissociating and denaturing protein complexes.
This gives the added advantage that linear protein strands will move more uniformly through the pores of the polyacrylamide gel. Some proteins are able to withstand the denaturing effects of SDS, which is why denaturing samples through heating in the presence of a reducing agent is also applied prior to carrying out an SDS-PAGE.
In order to carry out SDS-PAGE, a physical structure is needed to allow the process of protein separation. Two polyacrylamide gels are prepared:
- Stacking gel: Typically a low percentage polyacrylamide gel at pH6.8.
- Separating gel: Typically a higher percentage polyacrylamide gel at pH8.8.
Polyacrylamide provides the medium in which protein samples can be loaded onto an identical starting plane and then separate out, upon the activation of a constantly flowing electric current.
The concentration that the separating gel is cast at will allow for varying degrees of protein mobility. E.g. : a 15% gel would greatly limit the speed of separation of proteins in comparison to a 5% gel.
To create the gels, SDS, bisacrylamide, acrylamide, a buffer with an adjusted pH and a suitable stabiliser (like TEMED) are mixed together. The polymerisation takes place because bisacrylamide can form cross-links with 2 acrylamide molecules.
The ratio of the gel can therefore be modified to limit or increase cross-linking, which in turn affects the "pore" size through which proteins migrate inside the gels, thereby allowing for lesser or greater restriction on the migration of proteins.
E.g. A 10% acrylamide gel would slow the speed of separation of proteins whereas a 5% acrylamide gel would be less restrictive.
Higher percentage gels are therefore better for resolving low molecular weight proteins as they prevent proteins from running too quickly off the gel. Low percentage gels are particularly good for separating higher weight proteins because they allow a faster separation of proteins.
Protein samples are loaded into well pockets which are formed during the preparation of the stacking gel with the use of a plastic comb, which is removed after the gels have set.
Upon the application of a constant electrical field, the proteins migrate between two electrodes, away from the negatively charged cathode and towards the positively charged anode. In effect, the proteins move from the well into the stacking gel before lining up uniformly at the entrance to the separating gel where they then enter and separate in lanes through the gel.
There are 2 buffers needed to form an electrical circuit and for the protein separation procedure to succeed:
- Gel buffer: Tris-Cl based, is found inside the gel at pH6.8
- Running buffer: Tris-Gly based, is found outside the gel at pH6.3
Glycine is an essential component of each of the buffers and has a relative molecular charge of -1, 0 or +1. In its zwitterion format (no charge) both its amine and carboxyl groups are considered charged as +1 and -1, respectively.
Chlorine, on the other hand, is generally considered to be negatively charged in solution, thereby giving it a greater potential to move from the cathode toward the anode. As a component of the gel buffer, Chlorine has a greater potential than the Glycine found in the running buffer to move towards the anode.
There is a reason that the stacking gel, and the running gel have a different pH, and this is essential for the protein migration process. When glycine enters the stacking gel from the running buffer, the environment pH changes from pH8.3 to pH6.8.
This renders glycine to its zwitterionic state, and means that it moves very slowly in the negative current. But once the glycine reaches the running gel everything changes. The potential of the Chlorine ions in the running gel give what is called a “front”.
This front creates a narrow zone behind it, which creates a steep voltage gradient (a bit like a vacuum) in which the Glycine is dragged along. As the glycine migrates through the stacking gel it compacts all of the proteins from their wells within this narrow zone, and into a neat alignment called the "stacking effect".
Once the Glycine reaches the running gel the pH drastically increases from pH6.8 to pH8.8, which sees the glycine take on its negative conformation. So as the glycine front and the proteins all reach the running gel together, the glycine rapidly becomes anionic and races off down the gel, leaving the proteins to separate according to their molecular weight.