Related articles
Secondary antibodies resources
Alexa Fluor secondary antibodies
Biotinylated secondary antibodies
Enhancing Detection of Low-Abundance Proteins
9 tips for detecting phosphorylation events using a Western Blot
Western Blotting with Tissue Lysates
Immunohistochemistry introduction
Immunohistochemistry and Immunocytochemistry
Immunohistochemistry troubleshooter
Chromogenic and Fluorescent detection
Preparing paraffin-embedded and frozen samples for Immunohistochemistry
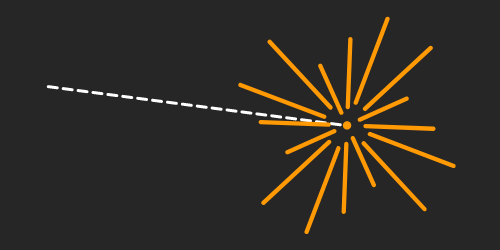
The Flow Cytometer
Principles of Flow Cytometry
One of the most valuable properties of the flow cytometer is its capability to measure both the physical and chemical characteristics of cells or particles.
When a sample is inserted into a flow cytometer, the particles contained within it are distributed down a funnel-like path known as the sample line, which has a wide enough diameter to allow the passage of various particles and cells.
This sample line causes them to line up into a single-filed stream, so they can be observed one by one by the laser beam detection system. The beam is scattered upon impact and the fluorescent light fragments are then counted and sorted or further characterized.
The Fluidics system
The actual alignment process is caused by an essential feature known as the fluidics system, which incorporates a central core surrounded by a sheath fluid. The sheath of the funnel through which the sample passes narrows towards the end, and the induced velocity forces streamlining of the sample by, what is known as, the Bernoulli effect.
This single stream of particles is called hydrodynamic focusing, and when laminar flow is sustained, the central fluid steam and the outside sheath fluid do not mix.
Detection, Lasers and Optics
After reaching successful hydrodynamic focusing, it is time to start interrogating the particles. To achieve that, all of them have to pass through at least one beam of focused light. Usually, that beam is produced by a laser.
Because of the ability to produce them with a specific wavelength and power levels, lasers are best suited for this job. They can range from ultraviolet all the way to the far red.
Forward scatter
When light is scattered forward after colliding with a particle, this results in around 20 degrees of offset from the beam’s axis. This light is then captured by either a photomultiplier tube or a photodiode and this process is called a forward scatter channel.
However, depending on the instrument you are using, the angle can be different. This technique can give an approximate particle size. Usually larger particles refract more light compared to the smaller ones, but the sample, collection angle, wavelength of the laser and sheath fluid all can impact the results.
What is a side scatter?
As the name suggests, when light is measured at a right angle to the excitation line, it is called a side scatter. This type of scattering gives us approximate complexity, like internal structures and the presence of granules. Similar to the forward scatter, it also depends on similar factors.
Both can be used at the same time to differentiate different cells in a mixture, but to obtain more details, fluorescent labelling is required.
What are the different types of filters and detectors?
When measurements are made at different wavelengths, qualitative and quantitative data is obtained about labelled surface receptors or intracellular molecules. Most modern cytometers have different channels and detectors for the emitted light, but their number varies from one instrument to the other.
The detectors can be photomultiplier tubes or avalanche photodiodes, but the tubes are the most common detectors. When a specific wavelength is required, filters are used. They can be organised in three major categories: long pass, short pass, and band filters.
The long pass optical filters allow only light above a specific length, cutting off everything under the stated number.
Short pass filters are essentially the same but reversed, allowing waves up to a specific length, stopping everything over that mark.
Band filters transmit only a bandwidth, which is a narrow range of wavelengths, hence the name band filters.
The dichroic filters are different. They can allow light to pass through them if it is over a specific wavelength and interfere with all the rest via a phased reflection.
The different functions of a dichroic filter
Apart from being a more sophisticated optical filter, it can also serve the role of a mirror. When placed at an angle to the incoming light, it can allow specific wavelengths to continue forward and reflect all the rest at a right angle, thus allowing the light to pass through numerous filters.
These filters allow multiple signals to be detected at the same time.
How are the signal and pulses processed?
When a particle goes through an interrogation point and creates a signal, the detector generates a pulse. When the particle enters the spot, where the laser beam is, it generates scattered light and fluorescence signals. The result is current from the anode of the PMT.
The number of protons interfering with the photocathode is proportional to the magnitude of the current and also to the intensity of both the scatter and signal from the particle. The pulses can be used in a graph by plotting the signal as a function of time.
How to quantify the pulse and remove unwanted signals?
The particle has entered the laser beam spot and currently it is in the centre of the laser beam and fully illuminated. This produces the maximum amount of optical signal and when the particle flows out of the beam, the current from the PMT gradually goes down to baseline.
What is a threshold setting?
When you have a specific particle, that you want to observe, keep in mind that not all signals correspond to it. In order to stop unwanted signals from processing, you have to decide what the signal intensity, known as a threshold, of the trigger channel should be.
Because PMTs are very sensitive and detect signals from all sorts of unwanted sources, it is necessary to have the threshold at an appropriate place, so non-essential data isn’t collected. If you don’t place a threshold, high background, masking relative data and overloading the detector may occur.
To do that, a parameter is set as a trigger for recording events. Commonly this is a forward scatter and any pulses that don’t exceed the desired threshold are ignored by all the detectors. If a signal is over that line, it gets fully processed.
Fluorescent markers and light
Fluorophores are used to detect the expression of nucleic acids, proteins, and other cellular molecules. These fluorescent markers gain light energy at a specific wavelength and emit it at a longer one. The process of accepting the energy is called excitation and the process of re-emitting the light is called emission.
Usually, emission happens a few nanoseconds after excitation and the process is known as fluorescence.
What is fluorescence?
When a fluorophore absorbs light energy, several things happen. First, its electrons go from resting to the maximal energy level. This level is called excited electronic singlet state and the energy needed to reach this state varies from one fluorophore to another.
This state ends rather quickly: typically, between 1 and 10 nanoseconds and then a conformational change takes place, leading to the electrons decreasing to a more stable level, known as the electronic singlet state. While reaching this state, some of the absorbed energy is released as heat.
After they reach the electronic singlet state, the electrons slowly continue to fall back to the resting state and release the leftover energy as fluorescence.
Should you use a fluorescent marker?
The reason why they are used is to directly mark the target epitope, so it can be measured. More than one fluorophore can be excited by the same laser, and multiple parameters can be measured simultaneously.